1. 서론
늘어나는 인류의 에너지 수요를 감당하기 위한 화석 에너지 사용 증가로 인류는 기후 변화(climate change)의 위기를 맞이하고 있다. 또한 불안정한 국제 정세로 인해 화석 에너지 가격의 급격한 상승까지 겹치면서 신재생 에너지에 대한 수요가 급증하고 있다. 여러 가지 신재생에너지 기술 중 태양광에너지(PV) 기술은 입사하는 태양광을 전기로 바로 변화하는 기술로 설치가 용이하고, 어느 공간에서도 발전이 가능하기에 설치가 급격하게 증가하고 있다. 2020년 기준으로 전세계 태양광 설치량은 773 GW를 능가하였고, 2025년 이전에 1 TW를 능가할 것으로 예상된다[1]. 또한 지속적인 기술개발로 인해 태양광의 균등화 발전가격(LCOE)이 미국 대규모 발전소 기준 0.05 $/kWh 이하로 내려가 화석연료 기반 에너지보다 가격 측면에서도 유리하다(Fig. 1(a))[2]. 이제까지 대부분의 발전소는 넓은 지역에 대규모의 태양광 발전소를 설치하여 대량의 전기를 생산하는 방식이었다. 향후는 가격 경쟁력을 갖춘 태양전지를 건물에 적용하여 에너지를 사용하는 건물일체형 태양광(BIPV), 차량일체형 태양광(VIPV) 등에 대한 수요도 급증할 것으로 예상된다[3-5]. 특히 BIPV 시장의 경우 급격한 시장 성장이 Fig. 1(b)와 같이 예상된다.
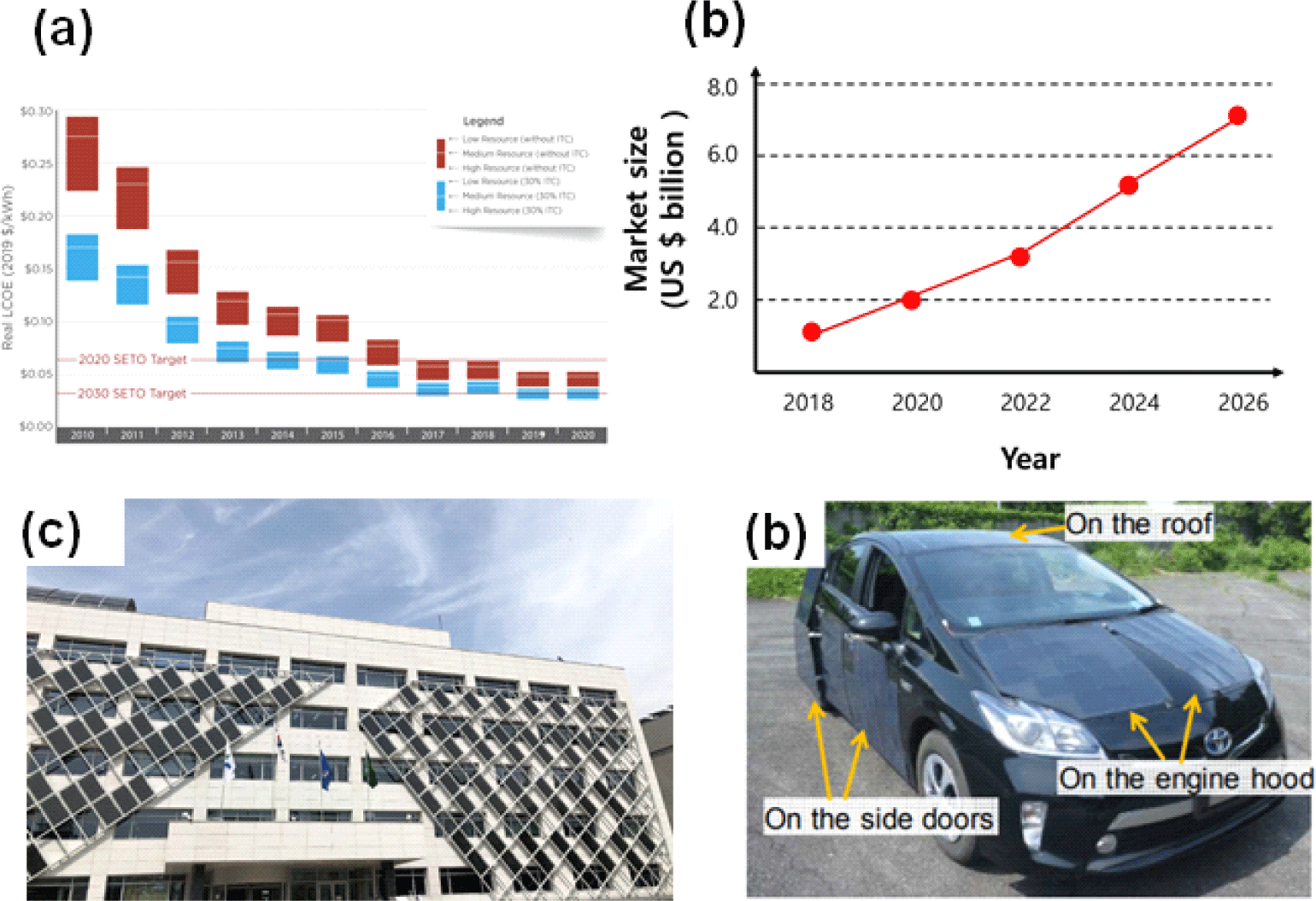
BIPV는 건물 외장재로 사용되기에, 높은 효율 이외에도, 심미성과 신뢰성 측면에 대한 개선이 필요하다. 다양한 보호 필름과 고온 고습에 강한 소자구조 도입을 통해 신뢰성의 문제는 해결하고 있다. 하지만 태양광의 심미성은 효율과의 Trade-off 관계로 인해 높은 효율을 유지하면서 컬러를 구현하는데 매우 어려움이 있다.
높은 효율을 구현하기 위해서는 가능한 넓은 영역에 입사광을 흡수해야 하기에, 고효율 태양전지 대부분은 검은색 색상을 나타낼 수밖에 없다. 하지만 검은색의 태양전지는 단조로운 색상으로 인해 태양광을 이용하여 건물이나 차량에 심미성 부여하는 데는 한계가 있다. 따라서 본 논문에서는 최소한의 입사광 손실을 통해 높은 효율을 유지하면서도 심미성을 강화하기 위해 제안된 인쇄 기반 컬러 태양전지 및 모듈 구현 방안에 대해서 소개하고, 이를 기반으로 향후 연구 방안에 대해 논의해 보고자 한다.
2. 인쇄 기반 컬러 태양광 구현을 위한 연구 방향
현재 제시된 인쇄기반 컬러 태양광 구현 기술은 Fig. 2와 같이 크게 4가지 방식으로 구분할 수 있다. 가장 많은 연구가 이루어진 부분은 태양전지 자체의 색상을 가지게 하는 방안이다. 주로 인쇄 공정을 미세하게 조정하여 태양전지 자체가 색상을 나타나게(optical engineering) 하거나, 인쇄가 가능한 광흡수 반도체 층의 밴드갭을 조정(bandgap engineering)하는 방식을 도용하였다. 이 경우, 색상을 가진 각각의 태양전지를 조합하여 컬러 태양광 시스템을 구성할 수 있다.
다른 한가지 방안은 검은색의 불투명(opaque)한 태양전지에 기능층을 도입하여 컬러를 구현하는 방식이다. 가능한 방식은 특정 파장의 빛만 선택적으로 반사(selective reflection)하는 다층구조의 반사막이나 나노 입자를 인쇄하는 방법이다. 다른 한가지 방식은 높은 에너지의 빛(자외선 혹은 청색광)을 이용하여 낮은 에너지로 변환하는 Down converting 효과가 나타나는 염료층을 인쇄하는 발광형 태양광(luminescent) 방식이다. 현재 컬러층을 도입한 기술의 경우, 본격적인 상업화를 앞에 두고 시제품들이 출시되고 있으며, 더 많은 연구를 통해 효율 감소를 최소화 하면서도 효율을 극대화하는 기술에 대해 연구 중에 있다. 각 방식에 구체적인 연구 방향은 아래와 같다.
3. 컬러태양전지를 이용한 컬러 태양광
컬러 태양전지는 태양광 모듈에서 가장 중요한 태양전지를 이용하여 다양한 색상을 구현하는 방식이다. 일반적인 태양전지는 양쪽 전극 사이에 광 흡수 반도체를 적용한 구조를 가지고 있다. 이때 태양전지의 두 금속 전극과 용액공정이나 건식 공정으로 광 흡수반도체 층 두께를 조절하면 cavity 효과 혹은 Fabry-Perot filter 효과를 통해 특정 파장의 빛만 선택적으로 반사하는 구조를 Fig. 3(a)처럼 구현할 수 있다. 이는 이미 디스플레이 산업에서 많이 사용되고 있으며, 효율 저하를 최소화하면서도 다양한 색상 구현이 가능한 태양전지를 만들 수 있다는 장점이 있다. 두 전극 사이에 유전체인 광 흡수층의 두께(d) 및 굴절률(n)에 따라서 특정 파장(λ)의 빛의 경로를 변경할 수 있다. 이때 peak가 되는 파장(λ)은 굴절률과 두께의 곱(n×d)에 의해 영향을 받는다. 따라서 인쇄 공정을 통해 굴절률 값을 알고 있는 광 흡수층 반도체의 두께를 제어하면 특정 색상이 반사되어 나와서 컬러를 구현하는 것을 Fig. 3(a)와 같이 알 수 있다[6]. Fig. 3(b)은 고분자(Polymer)층의 두께를 조절하여 다양한 색상을 구현한 예시이다. 그림에 나타난 것처럼 용액 공정 반도체의 농도, 인쇄 공정 등을 제어하면 쉽게 조절이 가능하다.
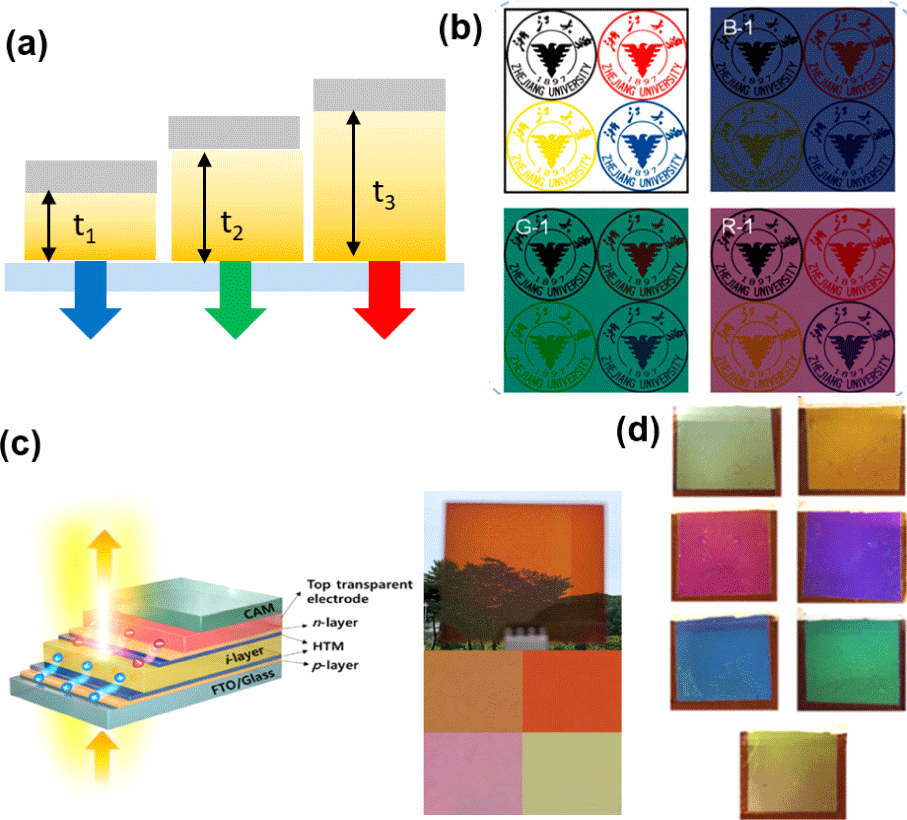
주로 이 방식을 사용하여 컬러를 구현하는 방식은 태양전지의 두께가 수백 nm 이하로 구성되는 박막 태양전지에서 많은 결과가 보고되고 있다. 이제까지 보고된 광 흡수층은 고분자 기반 유기물[6-8], 차세대 태양전지로 각광받고 있는 높은 효율을 나타내는 Perovskite[9-11] multi exciton generation이 가능할 것으로 예상되는 양자점(quantum dot)[12,13], 대면적 공정에 용이한 비정질 실리콘(amorphous silicon) [14,15] 등의 물질을 이용하여 제안되었다. 용액공정 기반 유기물 태양전지의 결과에 의하면 이 방식을 사용하여 10% 이내의 효율 손실로 청색, 녹색, 적색의 태양전지를 만들었다. 또한 투명 전극을 적용하면 Fig. 3(c)와 같이 색상을 구현할 수 있는 유리창을 만들 수 있는 장점도 있다.
이뿐만 아니라 광 흡수층 이외에도 태양전지를 구성하는 기판이나 전자 및 정공 수송층(electron and hole extraction layer)의 두께를 조절하여도 Fig. 3(d)와 같은 결과를 얻을 수 있다. 용액공정으로 형성된 Perovskite 태양전지에 두께가 다른 Meta surface 층을 도입하면 가시광선의 넓은 영역의 색상을 큰 손실없이 구현할 수 있는 장점이 있다[9,10,16,17]. 이 뿐만 아니라 전극 수송층으로 사용되는 TiO2 , SnO2 등의 두께 변화를 이용하여 선명한 색상의 고성능 태양광 시스템을 구축할 수 있다.
또한 CIGS 태양전지에서도 Buffer 층과 투명 전극 사이의 거리를 조절하면 다양한 색상의 빛을 반사하여 색상 구현이 가능하다. 투명 전극인 ITO와 buffer 층으로 많이 사용되는 ZnS, ZnO의 두께를 조절하여 조합을 형성하면 청색부터 노란색까지 색상을 구현할 수 있다[18]. 이 방식을 확대 적용하면 양면형 CIGS에도 색상을 부여할 수 있다[19]. CIGS의 태양전지의 경우, 신뢰성이 매우 우수하여 창호로 적용될 가능성이 매우 높다.
이와 함께 컬러 태양전지 구현을 위해 많이 고려되는 방안은 태양전지의 광 흡수 반도체 층의 밴드갭을 조정하여 특정 파장의 빛만 흡수하고, 나머지 빛은 통과시키는 방식이다. 반도체 물질은 물질 자체의 밴드갭보다 높은 에너지의 빛은 흡수하고, 낮은 에너지의 빛은 흡수 없이 통과시킨다. 이에 반도체 물질의 밴드갭을 조절하면 밴드갭보다 낮은 에너지를 가진 빛이 태양전지를 투과하여 색상을 나타낼 수 있다. 예를 들어 태양광 중 녹색 파장까지 흡수할 수 있는 반도체로 태양전지를 구현하면 붉은색 빛이 태양전지를 투과하여, 붉은색 빛으로 보이게 만들 수 있다. 예를 들어 Fig. 4(a)와 같이 특정 파장만 흡수하는 염료를 적용한 염료 감응형 태양전지(dye sensitized solar cell, DSSC) 의 경우, 염료가 흡수하는 색상 이외의 빛들은 모두 투과시켜 색상을 구현할 수 있다[20]. 이 방식의 가장 큰 장점은 건물 등의 투명한 창문 영역에 적용하여서 에너지를 생산할 수 있다는 것이다. 현재 기술에서는 인쇄 공정기반 컬러 DSSC를 건물 유리창에 적용하여 3% 이상 효율을 얻었으며, 수명이 20년 이상 확보된다면 건물에 널리 사용될 수 있을 것으로 예상된다[21].
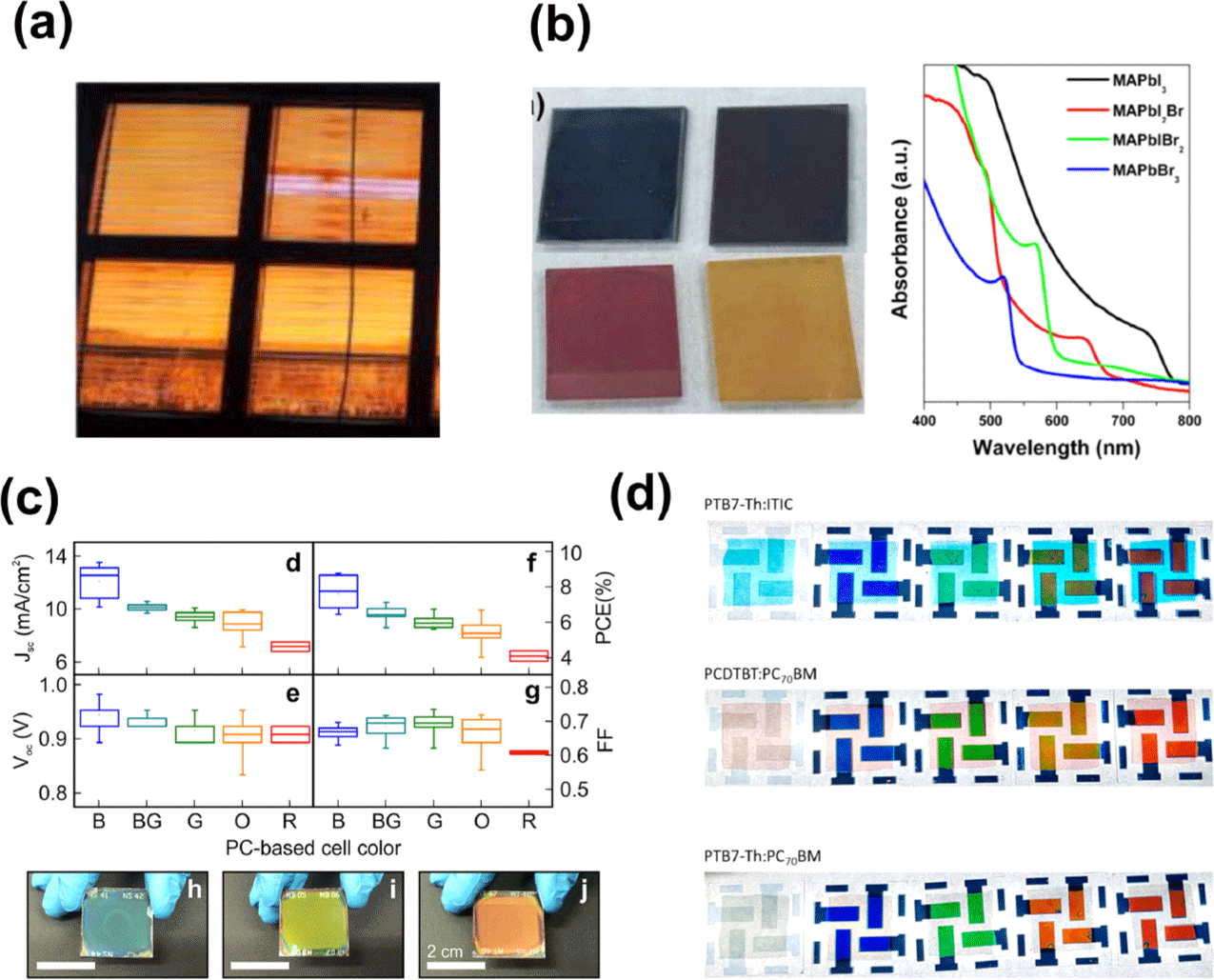
차세대 태양전지로 주목받고 있는 Perovskite 태양전지의 경우, 광 흡수 반도체 층의 halide 이온의 물질과 비율을 변경하면 밴드갭을 쉽게 조절하여 색상 구현이 가능하다[22-25]. Fig. 4(b)는 perovskite film에서 halide 이온인 Br과 I의 비율에 따른 색상 및 밴드갭의 변화이다[26]. Br의 비율이 높을수록 밴드갭이 넓어져 노란색 태양전지 구현이 가능하며, I 비율이 높을수록 검은색으로 색상이 변화한다. 이뿐만 아니라 유기 태양전지에서도 용액 공정이 가능한 다양한 고분자를 이용하여 특정 빛만 흡수하는 태양전지를 만들었다. 태양전지의 도너로 가장 많은 연구가 진행된 P3HT의 경우, 붉은색을 나타내며[27], PCDTBT 역시 적색의 태양전지를 용액 공정으로 구현하기 매우 유리하다[28]. 또한 도너 물질의 한 종류인 PTB7은 녹색의 태양전지를 구현하기 적합하고[29], 전자 받개 (acceptor)로 많이 이용하는 C60 대신 ITIC 같은 물질을 도입하면 효율이 높은 청색 태양전지 구현도 가능하다[30,31]. 이외에도 chalcogenide 기반 태양전지, 양자점 태양전지에서도 물질 구성의 변화 등을 통해 Bandgap을 조절하여 다양한 색상을 구현하였다.
태양전지의 색상을 더욱 뚜렷하게 만들기 위해서는 앞에서 제시된 두 가지 기술을 동시에 적용하는 방안으로 연구가 진행되고 있다. 용액 공정 반도체의 광 흡수 스펙트럼 조절을 물질 합성 및 조합을 통해 구현하고, 이에 두께 조절이 가능한 공정을 이용하여 Fabry-Perot filter 효과를 더하면 더욱 선명한 색상의 태양전지를 구현할 수 있다. Fig. 4(c)와 Fig. 4(d)는 perovskite, 유기 태양전지의 두 개의 기술을 동시에 적용하여 가시광 전 영역의 색상을 구현하였다[16,31]. 두 방식을 동시에 적용하면 청색부터 적색까지 가시광선 전 영역에서 선명한 색상 구현이 가능하여 원하는 색상으로 태양전지를 만들 수 있다. 이를 BIPV에 적용시 건물의 심미성을 상당히 향상시켜 줄 수 있다. 또한 건물의 유리창에 적용하기에 가장 최적화된 구조이다.
하지만 컬러 태양전지를 이용하는 방식의 컬러 태양광은 태양전지 출력 차이가 심하다는 치명적인 단점이 있다. Fig. 4(c)와 같은 태양전지에서는 청색 색상의 태양전지의 경우, 효율이 8% 이상 나타나는 반면, 적색의 경우 4% 이하로 효율이 2배 가까이 차이가 발생한다. 단일 색상의 태양전지를 직렬로 연결하여 태양광 모듈을 구현하는 경우에는 태양전지간 효율 차이가 발생하지 않아서 문제가 되지 않지만, 다양한 색상의 태양전지를 연결하는 경우 전류가 낮은 태양전지가 뜨거워지는 hot-spot이 발생할 수 있다[32]. 이에 색상별로 효율 및 전류 차이를 최소화하는 방향으로 연구가 진행되어야 한다. 또한 유리창의 경우 건축물의 생애동안 색상을 유지해야 하기에 신뢰성에 대한 추가 개선이 필요하다.
4. 컬러층 도입을 통한 컬러 태양광
한편, 태양전지가 결합된 태양광 모듈의 경우, 태양전지 이외의 부품도 많이 필요하다. 외부 충격으로부터 태양전지를 보호하기 위한 충전제(encapsulant) 와 전후면에서 기계 강도 및 외부 환경에 따른 변화를 막기 위한 (습도, 온도, 충격 등) 유리나 필름이 추가로 필요하다. 이 외에도, 전기적 연결을 위한 전선 및 회로 설비도 추가로 필요하다. 본 장에서 다루고자하는 컬러 기술은 모듈화 과정에서 필요한 재료들을 이용하여 컬러를 구현하는 방안에 대해서 다루고자 한다.
Fig. 5(a)와 같이 여러층의 유전체 박막 필름이 적층된 경우 박막 사이의 굴절률 차이로 인해 특정 파장의 빛을 보강간섭과 상쇄 간섭을 통해 반사시킬 수 있다. 이러한 원리는 여러가지 광 전자 소자 및 광학 소자에 많이 이용되어 반사 방지막(anti-reflection coating), 광 추출층 등에 널리 이용하고 있다. 태양전지에서도 이 기술을 적용하면 입사되는 다양한 가시광선 중 특정 파장만 선택적으로 반사하는 기능을 부여할 수 있다. 이 경우, 태양전지는 반사하는 빛의 색깔로 외부에서 인식되어 컬러 기능을 부여할 수 있다.
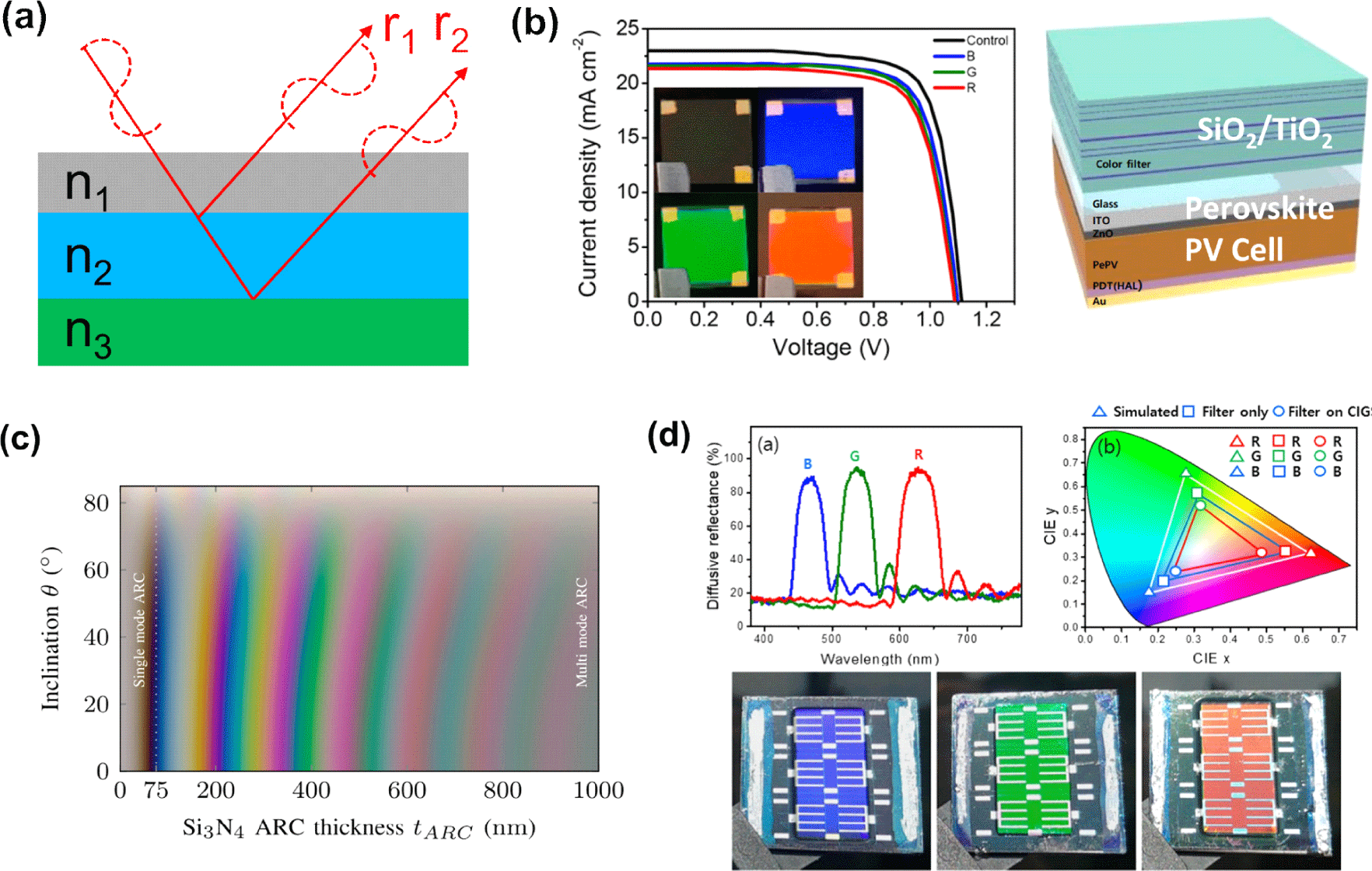
Fig. 5(b)∼Fig. 5(d)는 perovskite, 결정질 실리콘, CIGS 를 이용한 컬러 태양전지이다[33-35]. 각 연구의 경우, SiO2/TiO2, SiOX/SiNy, Al2O3/SiO2 층의 두께를 조절하여 다양한 색상을 구현하는데 성공하였다. 이 방식의 가장 큰 장점은 효율 저하가 적으면서도, 넓은 영역의 색상을 구현하기에 매우 적합한 방식이다. 특히 앞장에서 제시한 광학구조 및 밴드갭 조절 방식보다 더 많은 색상을 구현할 수 있으며, 불투명한 태양전지 전면에 적용할 경우 태양전지 색상과 무관한 색상들을 구현할 수 있는 장점이 있다. 또한 컬러층 도입에 의한 상대 효율 저하도 10% 이내로 고효율을 구현하는데 유리하며, 색상별 효율 차이의 문제도 극복할 수 있는 기술로 각광을 받고 있다. 앞에 제시된 박막이외에도 굴절율 차이가 있는 모든 박막에서 색상 구현이 가능하여, SiNx:H/Indium tin oxide, SiN/SiON, Si3N4/ ZnSe/a-Si 등의 조합으로 만들어진 적층 구조를 적용하여 색상을 구현하는 연구들을 제시하였다[18,36-38]. 또한 용액공정이 가능한 TiO2, SnO2 등을 이용하여서도 선택적 반사막 구현이 가능할 것으로 예상된다[39]. 색상 구현을 위해 사용되는 막은 주로 무기물로 구성되어서 고온이나 습도에 대한 신뢰성이 매우 우수한 장점도 있다.
반사되는 빛의 파장이 박막 두께에 따라 변화하기에 매우 정밀한 두께 조절이 필요하다. 이로 인해 고가격의 진공 증착 중심으로 연구 개발이 이루어져서 선택적 반사막을 적용한 컬러 태양광의 가격 경쟁력 확보에 어려움이 있다. 인쇄 공정의 경우, 박막 형성 공정이 가격 측면에서 진공 증착 방식 대비 장점을 가지고 있음에도 불구하고, nm 단위의 두께 조절의 어려움으로 제품화로 연결되지 못하고 있다. 향후 정밀한 인쇄 공정이 개발된다면 컬러 모듈 시장 경쟁력 확보에 큰 기여를 할 수 있을 것이다. 또, 하나의 극복해야할 문제점은 각도의존성이다. 입사하는 빛의 각도나 관찰자의 위치에 따라 반사되는 빛의 파장이 변화하여 정면에서 나타나는 색상과 측면에서 나타나는 색상의 차이가 발생하는 문제점이 지적되고 있다.
이를 해결하기 위해 제안된 방안들은 Fig. 6과 같다. 먼저 각도 의존성을 낮추기 위해서 태양광 모듈의 가장 상부에 있는 층에 요철을 적용하는 방식이다[40,41]. 요철을 적용하게 되면 반사된 빛의 방향성을 낮추는데 기여할 수 있어, 각도에 따른 색상 의존성 문제를 해결하는데 기여할 수 있다. 인쇄 공정은 다양한 투명한 구조체를 기판에 형성하는데 매우 적합하기에 선택적 반사막을 이용한 컬러 태양전지에 각도 의존성을 줄이는데 인쇄 공정이 크게 기여할 수 있을 것으로 예상된다. 다른 방식은 나노 입자나 구조체를 이용하여 각도에 상관없이 색상을 구현하는 방식이다 [42,43]. 주기를 가지고 인쇄된 전극이나 나노 입자는 특정 파장의 빛과 공진(resonance)하여 색상 구현이 가능하다. 발전된 인쇄 공정을 통해 대면적화에 성공한다면 이러한 기술을 이용한 컬러 태양광에 대한 수요가 증가할 것으로 예상된다.
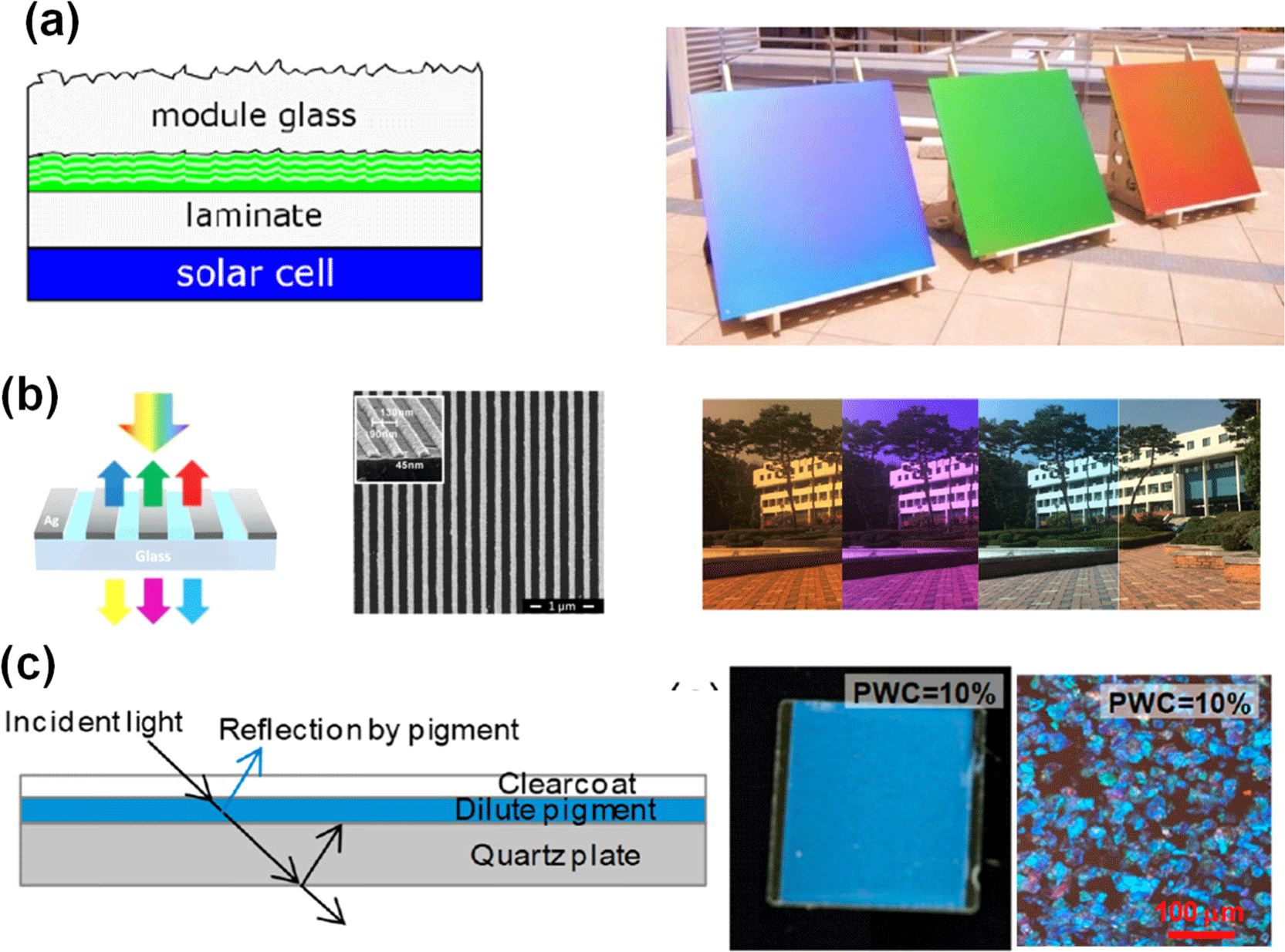
한편, 일본 Toyota사를 중심으로 기존에 자동차의 빛을 반사하는 안료로 많이 쓰이는 운모를 기반으로한 용액 공정용 반사체(mica)를 이용하여 컬러 태양광을 구현하는 방식에 대해 많은 연구가 이루어지고 있다 [4,44]. 선택적 반사막을 하나의 막으로 형성하는 것이 아니라, 반사가 가능한 마이크로 사이즈의 입자들을 용액에 넣어서 인쇄 공정으로 반사막을 구현하는 방식이다. 이러한 공정들은 선택적 반사막 기반 컬러 태양광의 단점들을 극복해 줄 수 있을 것으로 기대된다. 또한 사용가능한 무기 입자들의 신뢰성이 충분히 확보되어 있기에 시장 진입에 큰 어려움이 없을 것으로 예상된다.
마지막으로 소개할 기술은 선택적 반사막의 경제성 및 성능 확보를 위한 반사막을 특정 부분에만 인쇄하는 방식이다(Fig. 7 (a)) [45,46]. 특정 파장 빛만 반사할 수 있는 무기물 기반 반사층을 태양광 모듈의 전면이 아닌 일부 부분에만 인쇄를 통해 막을 형성한다. 이 경우, 인쇄가 된 영역으로 입사하는 빛의 일부는 외부로 다시 나가서 색상을 구현하고, 나머지 파장의 빛이나 나머지 영역에 입사되는 빛은 손실없이 태양전지로 전달될 수 있다. 관찰자가 멀리서 보는 경우, 일부분에 인쇄된 반사체에 의해 전체가 색상을 띄는 것처럼 보이게 된다. 물론 이 방식도 Fig. 7 (b) 및 Fig. 7(c)에 나타난 것처럼 반사층이 전면 유리의 넓은 영역에 인쇄되는 경우 투과도가 감소하여 효율 감소가 나타나지만, 적절한 간격 조절을 통해 효율을 극대화할 수 있는 장점이 있다. 따라서 시장에서 수요가 급증하고 있으며, Fig. 7(d)의 예시와 같이 (서울에너지공사 방음벽, 서울시 양천구 소재) 일부 제품들이 실제로 적용되어 신뢰성과 성능에 대한 평가를 받고 있는 상황이다.
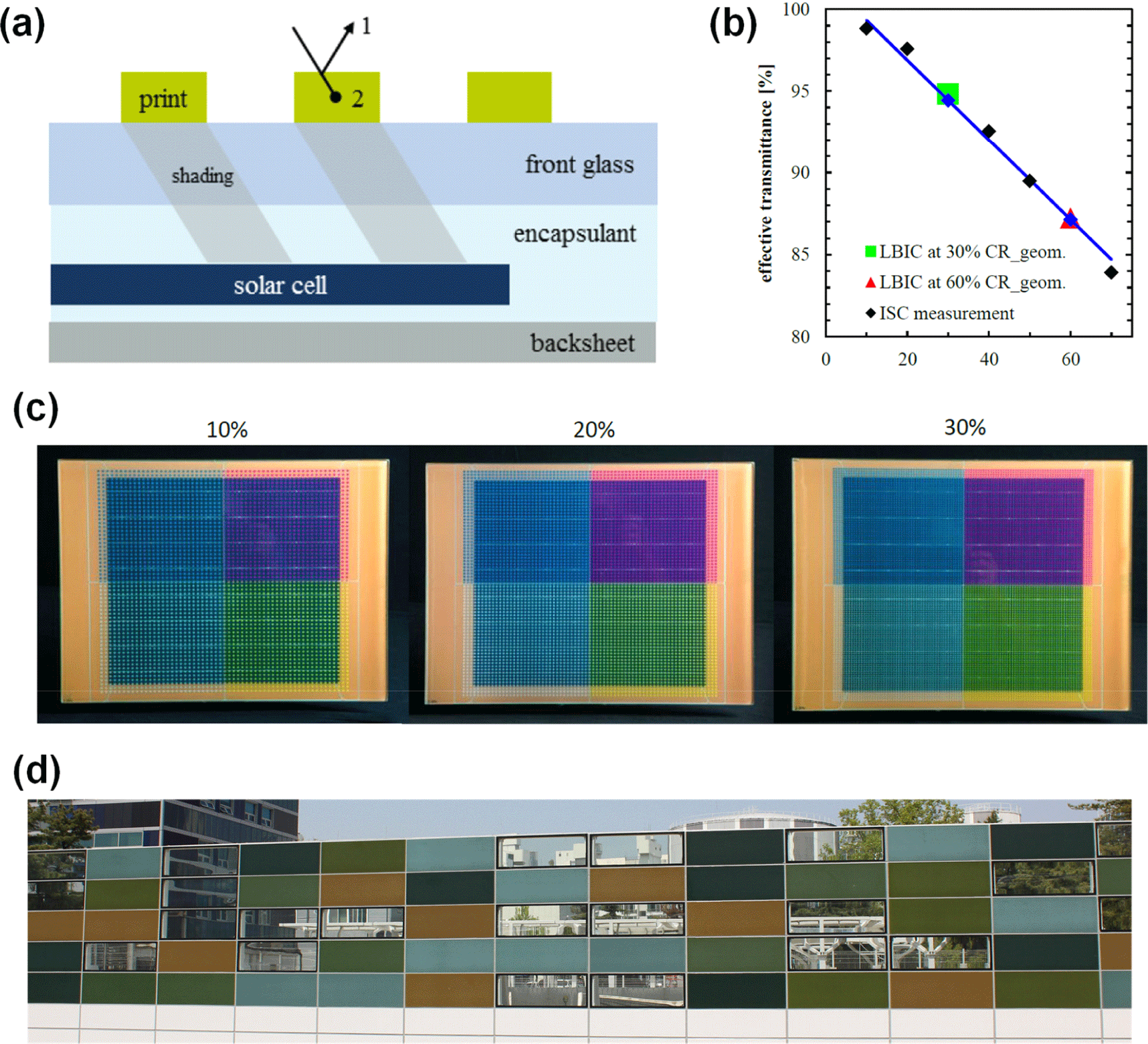
선택적 반사막 이외에 보호유리, 필름, 봉지재 등을 이용하여 색상을 구현하는 방식은 발광체(luminophore)를 태양광 모듈에 적용하는 방식이다. Fig. 8(a) 와 같이 유기물이나 Core/shell 구조의 양자점에서는 밴드갭보다 높은 에너지의 빛을 흡수하여 밴드갭보다 낮은 에너지를 발광하는 Down-converting 현상이 발생한다. 이를 이용하면 입사하는 태양광의 높은 에너지 빛을 흡수하여 다양한 색상을 구현할 수 있다[47,48]. 즉, 자외선이나 청색광을 흡수하여 녹색, 노란색, 적색 등의 색상을 나타내는 유리 구현이 가능하다. Fig. 8(c)에 나타난 것처럼 고분자의 구조나 양자점의 크기를 조절하면 흡수 파장과 재발광 빛의 파장을 조절할 수 있다. 이때 태양전지의 색상의 재발광하는 빛의 색상에 따라 변화하기에 이러한 구조의 컬러 태양광을 컬러 태양광으로 정의할 수 있다.
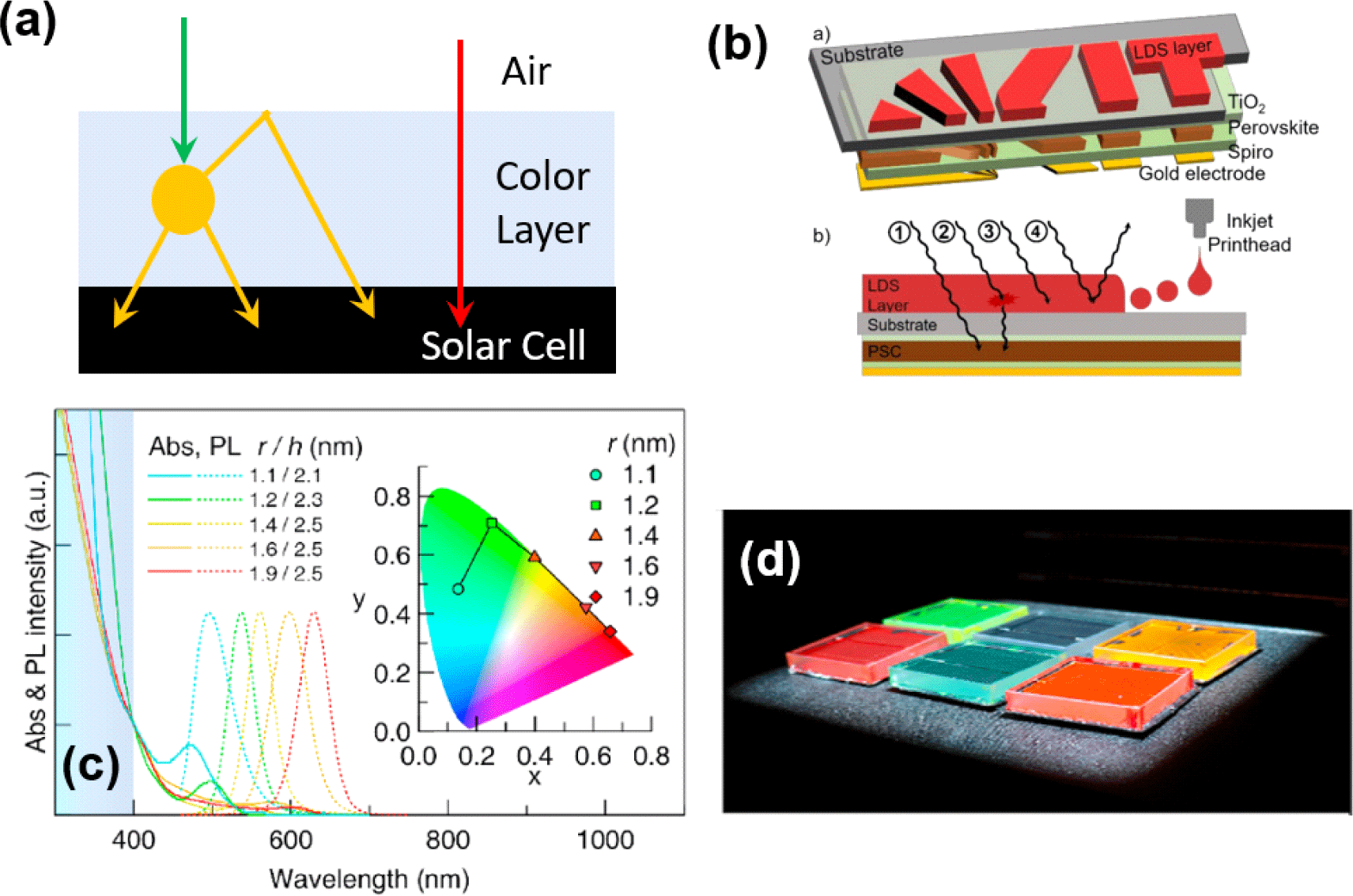
본 공정의 가장 큰 장점은 발광체의 색상에 따라서 색이 결정지어지기에, 발광체의 색상만큼 다양한 색상을 구현할 수 있는 장점이 있다. 또한 태양전지가 잘 사용하지 않는 높은 에너지의 자외선과 청색광을 이용하여 색상을 구현하기에 색상 구현에 따른 효율 저하를 최소화할 수 있다[49]. 그리고 인쇄공정과 결합한 경우, 다양한 그림이나 문자를 태양전지 전면에 인쇄 가능하다는 장점이 있다[48,50]. 또한 발광층 형성 역시 인쇄 공정을 이용하기에 가격 경쟁력 측면과 대면적화 측면에서 다른 기술 대비 장점이 있다. 하지만 아직 발광체의 열과 빛에 대한 안전성이 충분히 검증되지 못한 문제점이 있어, 향후 발광체의 신뢰성이 개선된다면 시장 진입에 매우 유리할 것으로 예상된다.
5. 결론 및 전망
본 논문에서는 현재까지 연구와 개발이 이루어지고 있는 컬러 태양광의 기술에 현황에 대해서 알아보았다. 컬러 태양광의 도심형 태양광에 대한 수요 증가로 인해 BIPV 및 이동형 태양전지에 많이 사용될 것으로 예상된다. 현재까지 제시된 기술은 크게 태양전지 자체가 색상을 가지거나, 태양전지 전면에 기능층을 도입하는 방식으로 이루어지고 있다. 태양전지 자체가 색상을 구현하는 방식은 흡수층을 포함한 태양전지의 두께 변화 및 흡수층의 밴드갭 조절을 통해 컬러 태양광을 구현하고 있다. 반면, 기능층을 도입하는 방식은 태양광 모듈의 전면 유리, 충전재, 보호 필름 등에 기능층을 형성하는 방식을 적용한다. 이때 기능층은 특정파장만 반사하거나, 빛을 재발광하는 방식을 이용한다. 많은 인쇄 공정이 컬러를 구현하는 기술에 적용되고 있으며, 기술이 고도화되는 경우 인쇄 기술을 기반으로 한 컬러 태양광의 시장진입이 이루어질 것으로 예상된다.