1. INTRODUCTION
All inorganic CsPbX3, where X is Cl, Br, or I, perovskite nanocrystals (CPX NCs) have gained immense interest because of their exceptionally high photoluminescence (PL) quantum yield (QY) [1,2]. Defect-tolerant properties resulted from the presence of Pb element make them not to need surface passivation to achieve high PL QY [3,4]. In addition, mass-producible simple colloidal synthesis, solution processibility, and ease of band gap tuning enabled by composition engineering make the CPX NCs highly attractive in various optoelectronic applications such as light emitting diodes (LEDs) [4-7], photovoltaic cells [8,9], and detectors [10].
However, further progress of CPX NC-based optoelectronic devices have been retarded due to instability of CPX NCs such as merging [11], easy desorption of surface ligands [12] and thermal degradation [13]. For example, high PL QY of CPX NCs rapidly diminishes since nanometer-sized domain of CPX NCs become larger by merging process [11]. When the domain size become larger, radiative recombination of charge carriers is hindered, resulting in decrease of PL QY [17]. Also colloidally synthesized CPX NCs easily decompose due to weak binding of ligands on surfaces [12].
Recently merging and ligand desorption issues could be notably alleviated by development of surface engineering of CPX NCs [12,14,15]. Removal of merging-vulnerable facets was effective for suppression of merging, and introduction of new ligand system enhanced binding energy on surfaces [16]. However, thermal instability issue, which should be overcome for realization of optoelectronic devices working at high temperature, has not been addressed clearly.
Here, we demonstrate remarkably enhanced thermal stability of CPX NCs. Colloidal CPX NCs prepared with the presence of impurity metal halides showed high thermal stability with markedly suppressed merging and phase transformation while CPX NCs synthesized without impurity metal halides exhibited drastic merging and phase transformation at elevated temperature. By investigating various impurity metal halides, we could extent the list of metal halides which are effective for enhancement of thermal stability. Finally we proposed mechanism on enhancement of thermal stability of CPX NCs.
2. METHODS
For the synthesis, cesium oleate solution was prepared by mixing 2.5 mmol of cesium carbonate, 2.5 mL of oleic acid (OA) in 40 mL of 1-octadecene (ODE). The temperature was set to 100°C for degassing, and then the cesium oleate solution was kept at 150°C before injection.
CsPbX3 (CPX where X is Br or I) NCs were synthesized basically following the previously reported procedure with some modifications [1,11]. First, 3.76 mmol of PbX2, 10 mL of OA, and 10 mL of oleylamine (OLA) were mixed in 100 mL of ODE. Then the solution was degassed for 2 h at 100°C. Next, temperature of the solution was raised to 170°C under Ar flow, and 8 mL of cesium oleate solution was swiftly injected. After 10 s, the reaction flask is rapidly cooled to room temperature by immersing in a water bath. For the synthesis of CPX under the presence of impurity metal halides, 4.2 mmol of impurity metal halides (e.g., ZnX2, SnX2, InX3, and GeX2) were introduced in the flask containing PbX2, OA, and OLA, and other procedures were conducted in same manner with the synthesis of pristine CPX NCs.
The synthesized NCs were purified by centrifugations. The reaction crude solution was centrifuged at 10,000 rpm for 10 min. And then the precipitate was redisperesed in toluene, and centrifugation was performed again (13,000 rpm for 30 min). The resulting precipitate was redispersed in hexane, and the solution was centrifuged at 6,000 rpm for 10 min. Finally, the supernatant was collected and filtered using syringe filter. The resulting NCs were stored under inert conditions.
For thermal stability test, CPX NCs were deposited on glass substrates by drop casting. After deposition the CPX NC films were placed on a hot plate at desired temperature for thermal stability. We note that all the thermal stability tests were performed in inert conditions.
3. RESULTS AND DISCUSSION
Fig. 1(a) shows absorption and PL spectra of CsPbBr3 NCs synthesized based on previously reported procedures without impurity metal halides (pristine-CPB NCs) [1]. The PL peak position is around 512 nm (green color, see the inset in Fig. 1(a)) with very narrow full width at half maximum (FWHM) of 21 nm possibly due to very weak quantum confinement effect resulted from their larger size than exciton Bohr size [2]. From analysis using transmission electron microscopy (TEM), it was confirmed that fresh pristine-CPB NCs are cube shape with average size around 10 nm, which is in accordance with earlier studies [2], and they were individually well distributed on a TEM grid (Fig. 1(b)).
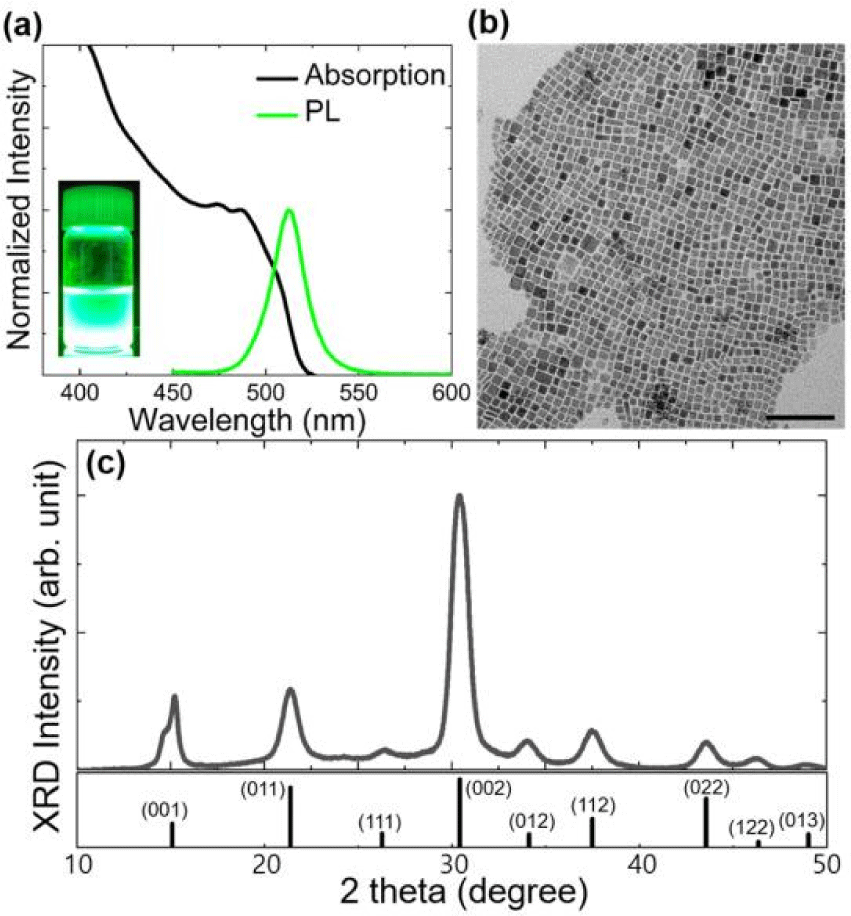
XRD patterns were collected using a CPB NC film on a glass substrate, and the XRD patterns revealed the crystallographic structures of CPB NCs (cubic phase) as shown in Fig. 1(c). Patterns were noticeably broad due to nanometer-size of CPB NCs [11,13].
Thermal stability of CPB NCs is a very important property because the temperature of various optoelectronic devices (solar cells, LEDs, and detectors) rises during operation. Therefore, in order to investigate the thermal stability of CPB NCs, we heated CPB NC films. After heating for 1 h at 200°C, the XRD patterns of pristine-CPB NCs were significantly sharpened due to increased size (Fig. 2(a)). Direct visualizations based on TEM analysis confirmed markedly enlarged structures of pristine-CPB NCs after heating. Approximately 10 nm average size increased (Fig. 2(b)) to ~300 nm after thermal stability test as shown in Fig. 2(c).
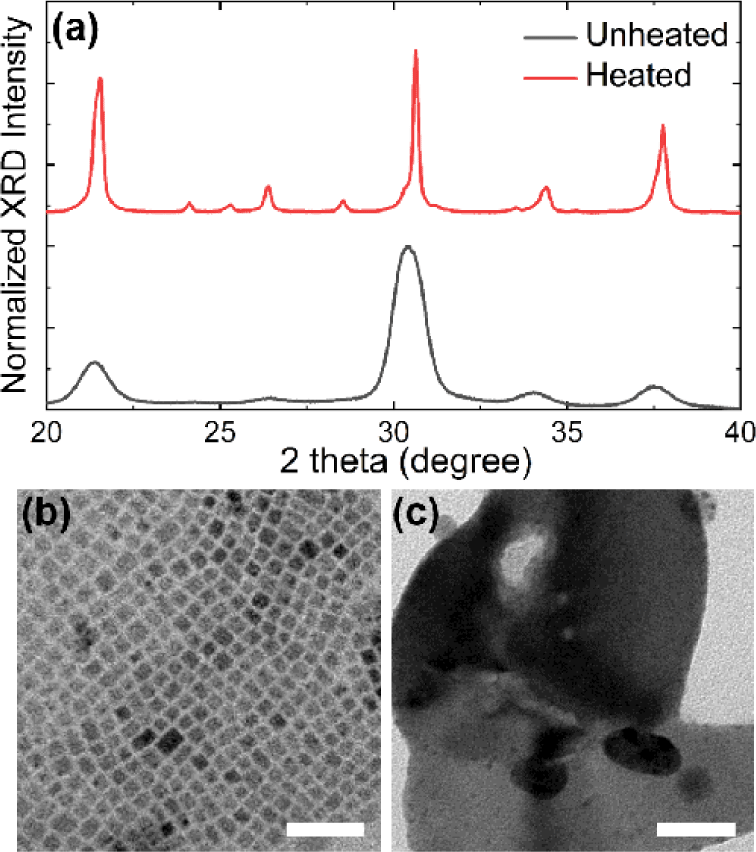
Previously it has been reported that CPB NCs merge under air condition [11]. Since PL QY drastically decreases upon merging of CPB NCs due to dissociation of charge carriers [17], merging of the NCs must be prevented to fabricate high-performance light-emitting devices. It was suggested that lacking of steric hindrance on surfaces should lead to the formation of directionally merged structures of CPB NCs [16]. We believe similar mechanism could play in merging of CPB NCs after heating.
We postulated that thermal stability of CPB NCs against merging can be improved by synthesizing the NCs with surface structures having less exposed (110) surfaces because (110) surface was known to be the site where merging exclusively initiates due to lack of steric hindrance, indicating absence of organic ligands [16]. One of the way to effectively synthesize the CPB NCs without (110) surfaces is adding impurity metal halides during the synthesis. When impurity metal halides present during the synthesis, oleylammonium- passivated CPB NCs can be formed. At the same time, exposure of (110) surface can be highly suppressed since formation of (110) surface becomes energetically unfavored [16]. Therefore we explored the thermal stability of CPB NCs synthesized with addition of impurity metal halides (e.g., ZnBr2) as shown in Fig. 3.
As shown in Fig. 4(a), XRD patterns of as-prepared CPB NCs synthesized under the presence of ZnBr2 (ZnBr2-CPB NCs) are very similar compared to XRD patterns of as-prepared pristine-CPB NCs. However, the results were in stark contrast after thermal stability test. While XRD patterns of pristine-CPB NCs become significantly sharpened (Fig. 2(a)), the XRD patterns of ZnBr2-CPB NCs showed nearly no change even after heating, indicating merging of NCs was effectively suppressed (Fig. 4(a)). Changes of peak ratios after thermal stability test in XRD patterns can be attributed to rearrangement of NC ensemble after heating. TEM analysis clearly demonstrated almost-perfectly suppressed merging after thermal stability test at 200°C (Fig. 4(b) and Fig. 4(c)). We note that ZnBr2-CPB NCs were also stable even for prolonged heating time (Fig. 5(a)). After heating, PL QY of ZnBr2-CPB NCs could be retained much better compared to PL QY of pristine-CPB due to effectively suppressed merging in ZnBr2-CPB NCs (Fig. 5(b)). It is noted that Zn element was not detected in ZnBr2-CPB because Zn-related species were removed during purifications due to absence of direct coordination (Fig. 5(c)).
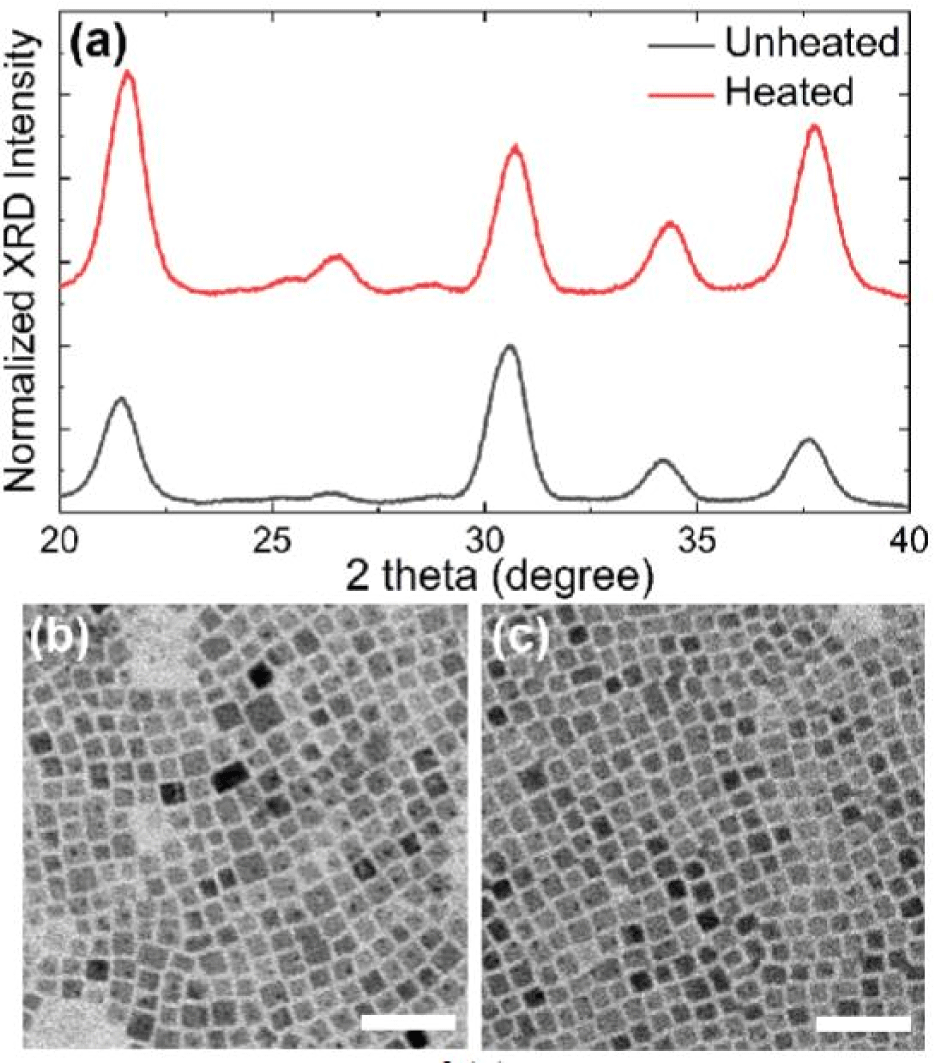
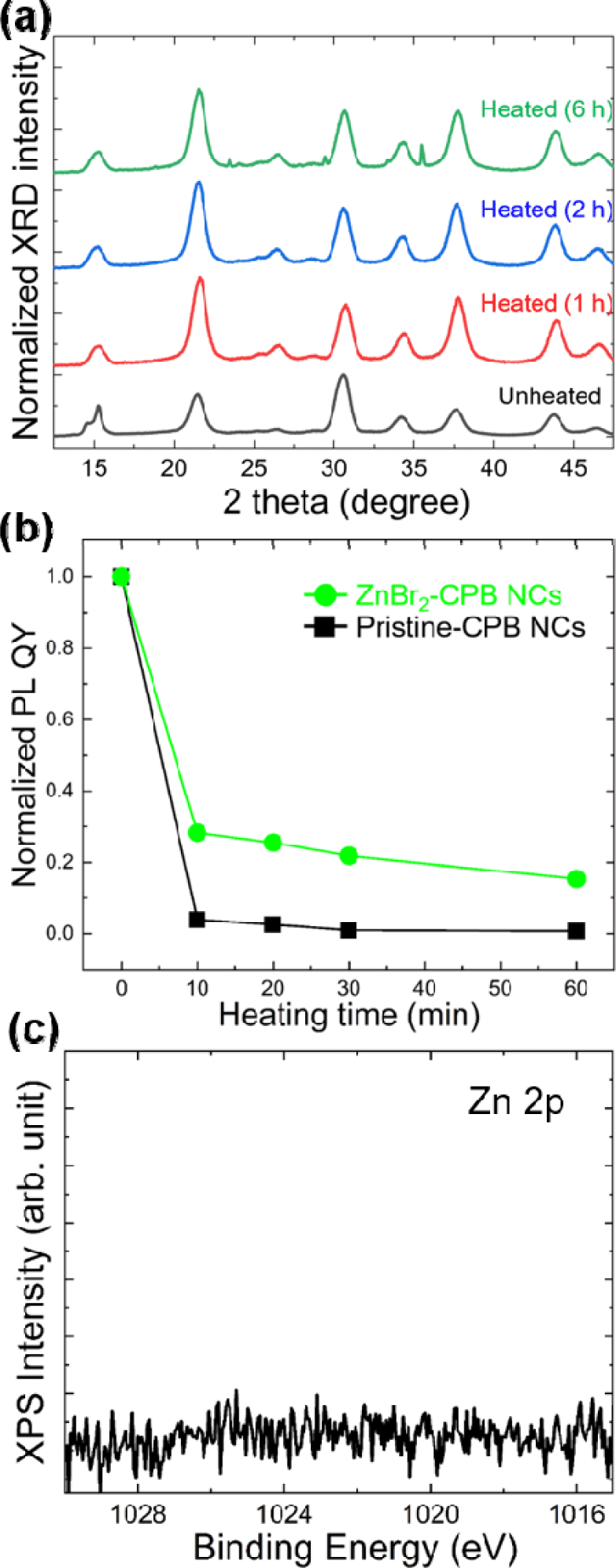
In order to investigate the effect of other metal halides, CPB NCs were synthesized with addition of various metal halides such as SnBr2, InBr3, and GaBr2 (The resulting CPB are denoted hereafter as SnBr2- CPB, InBr3-CPB, and GeBr2-CPB, respectively). SnBr2-, InBr3-, and GeBr2-CPB NCs showed XRD patterns of cubic phase CsPbBr3 crystals (Fig. 6). Importantly, thermal stability was considerably improved in all SnBr2-, InBr3-, and GaBr2-CPB NCs, and the fact was clearly demonstrated in broad XRD patterns of CPB NCs even after heating at 200°C (Fig. 6). With the best of knowledge, this is the first demonstration of significantly enhanced thermal stability in CPB NCs synthesized with various impurity metal halides.
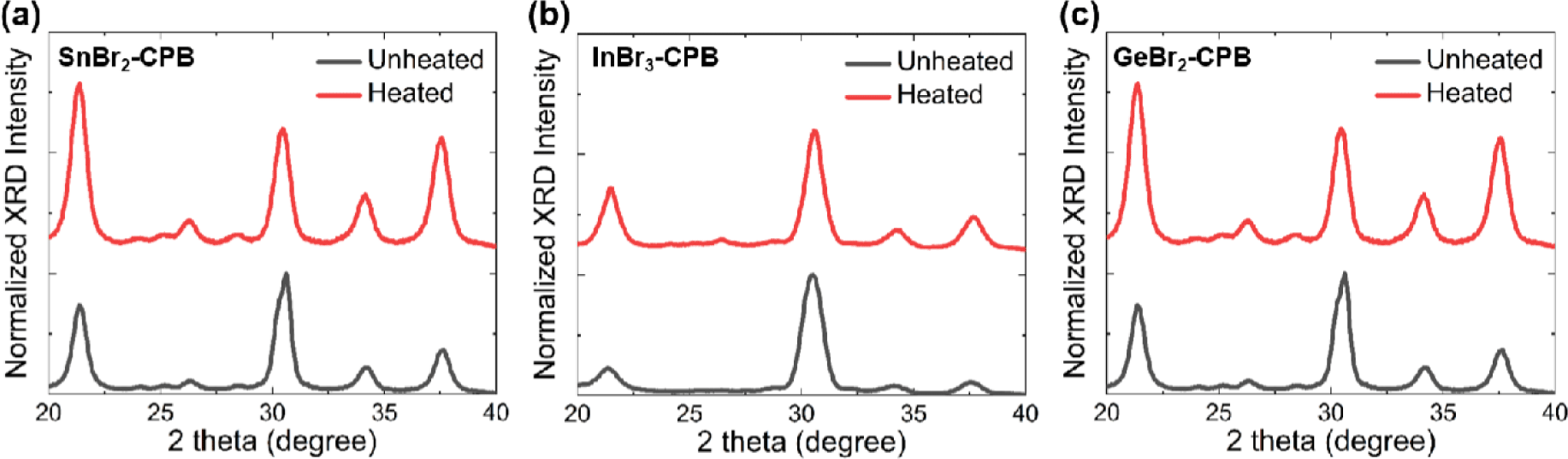
Based on experimental evidences, we propose mechanism on enhancement of thermal stability in CPB NCs prepared using impurity metal halides (Fig. 7). Similar to earlier studies, which demonstrated merging process occurred under ambient condition, presence of (110) surface may have much to do with merging, and the merging should be a heat-driven process. However, merging can be strikingly suppressed by minimizing the exposure of (110) surfaces of CPB NCs, and such thermally stable surface structures can be obtained by introducing various metal halides during the synthesis of CPB NCs.
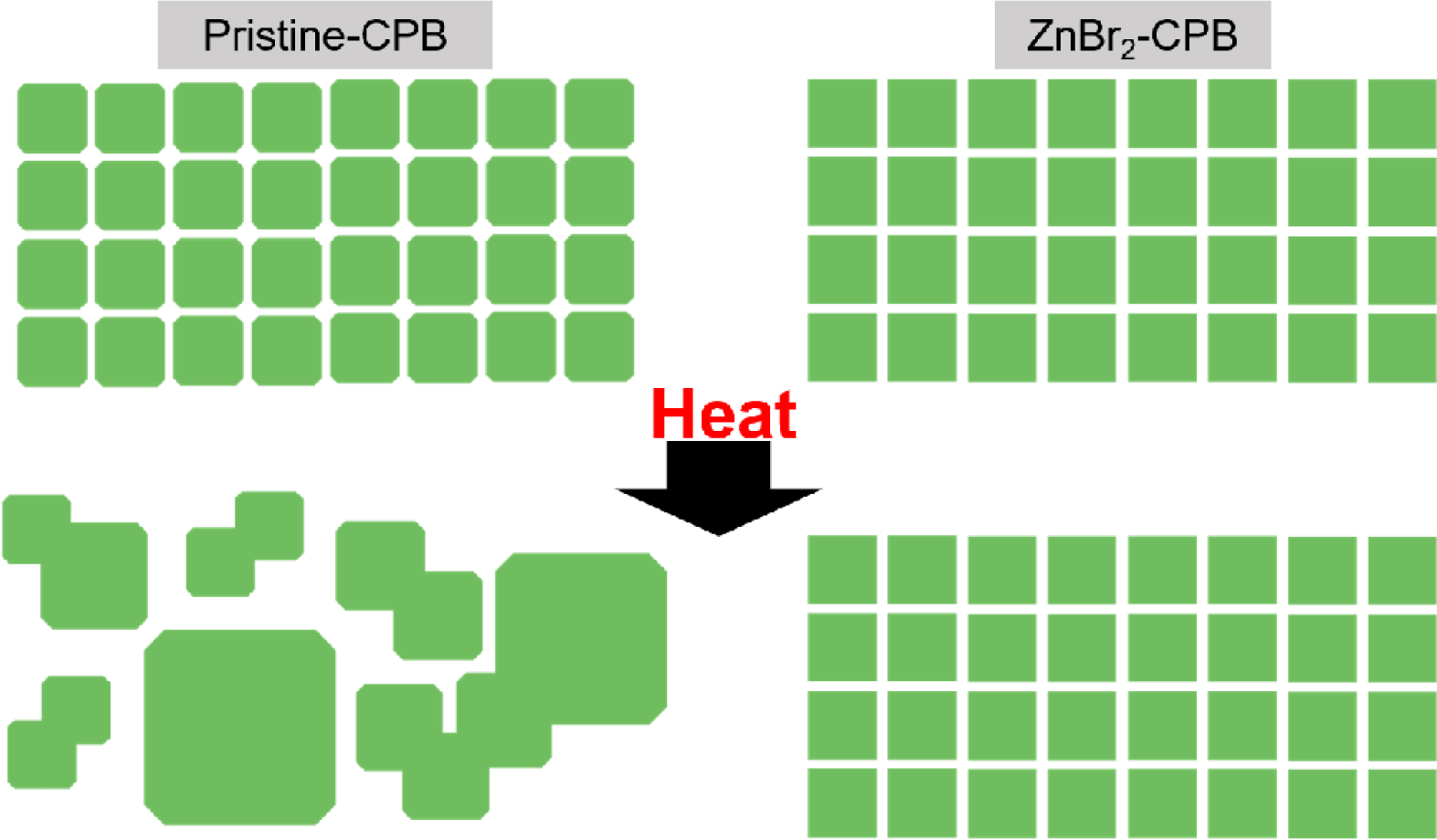
In addition to instability on merging of CPB NCs, one of the most widely reported instability in perovskite NCs is phase instability observed in CsPbI3 (CPI) NCs. Although cubic phase (black phase) is highly desired in CPI NCs, the cubic phase easily transforms to undesired orthorhombic phase (yellow phase) under various stimuli [9,18,20]. Therefore, to test the effect of metal halides on thermal stability of CPI NCs, we synthesized CPI NCs with or without impurity metal halide (e.g., ZnI2).
As reported in many previous studies, cubic phase of as-prepared pristine-CPI NCs was very unstable [9,20]. Initially obtained cubic phase rapidly transformed to orthorhombic phase upon heating as clearly evidenced in XRD patterns of pristine-CPI NCs (Fig. 8(a)). However, surprisingly, cubic phase of ZnI2-CPI NCs was very well retained, as shown in Fig. 8(b), corroborating the fact the phase stability of CPI NCs was strikingly improved by adding impurity metal halides during synthesis.
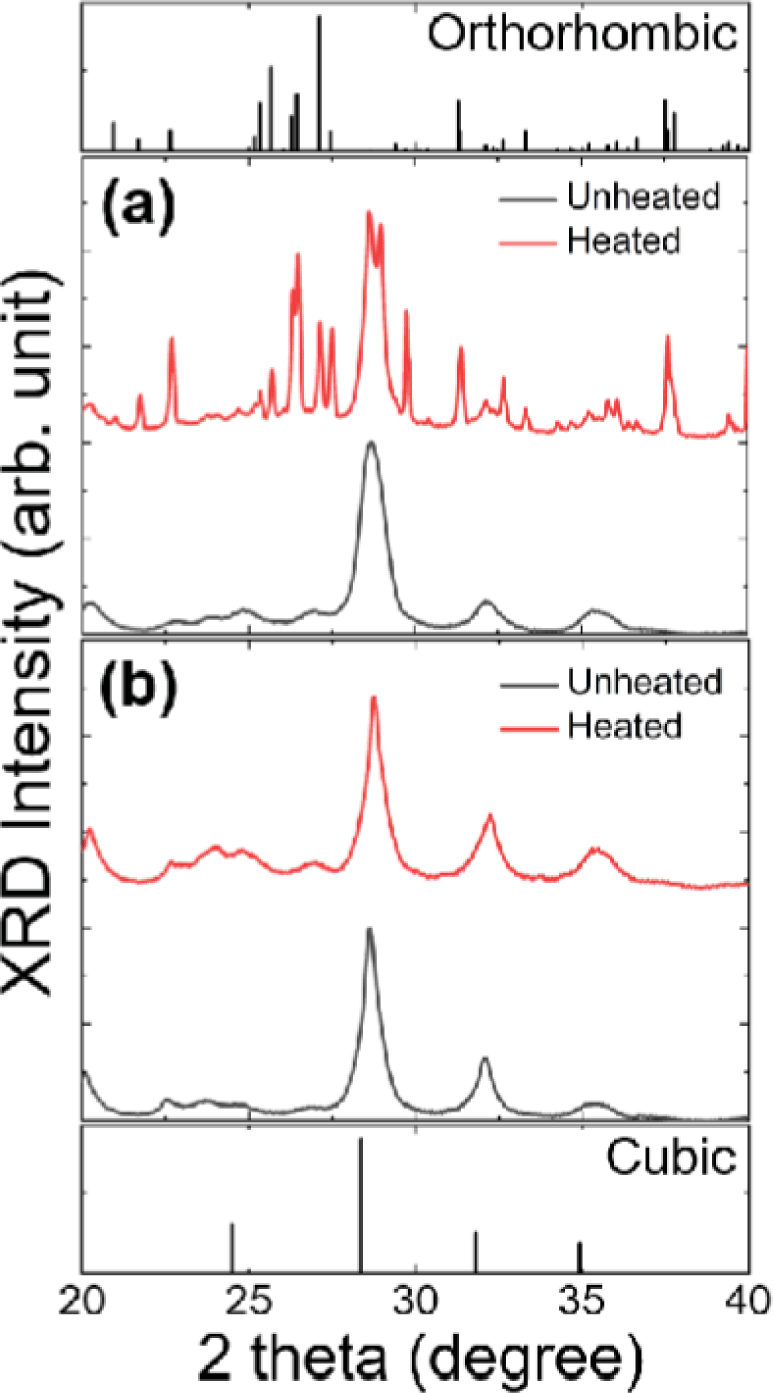
4. CONCLUSION
In conclusion, we investigate the thermal stability of all inorganic CPX NCs (X=Br, I). CPB NCs synthesized through a typical hot-injection method exhibited poor thermal stability. The NCs significantly merged upon heating at elevated temperature. However, addition of impurity metal halides (e.g., ZnBr2, SnBr2, InBr3, and GeBr2) effectively help to enhance stability against merging. And the enhanced stability against merging could be interpreted in suppressed formation of (110) surfaces, which have nearly no steric hindrance, under the presence of impurity metal halides. Same strategy also worked very effectively to enhance the phase stability of CPI NCs. Cubic phase of CPI NCs synthesized with impurity metal halides become very stable against heating. We believe our work on enhancing the thermal stability of CPX NCs provide new insight to prepare high performance optoelectronic devices based on CPX NCs with prolonged operating lifetime.