1. INTRODUCTION
Organic electrochemical transistors (OECTs) utilize ion injection from an electrolyte to control the bulk conductivity of an organic semiconductor channel [1]. The interaction between ionic and electronic charges throughout the entire channel volume gives OECTs superior transconductance compared to field-effect transistors. The tunability of their synthesis, ease of deposition, and biocompatibility of organic materials make OECTs particularly well-suited for applications in biological interfacing, printed logic circuits, and neuromorphic devices [2-5]. This suitability arises from the properties of organic mixed ionic-electronic conductors (OMIECs), which are soft electrical (semi-) conductors capable of efficiently supporting both ionic and electronic transport [6].
In polymer based OMIECs, the distinction lies between polyelectrolytes and polymer electrolytes. OMIECs with ionic charge have a stable ionic moiety that may be accompanied by a counterbalancing ion, a stabilized electronic charge on a conjugated segment, or exist as a self-balanced zwitterion. Conversely, some OMIECs are not inherently charged but contain polar moieties that can solvate ions. In these non-charged OMIECs, ionic species are incorporated physically during deposition or through contact with an electrolyte. Conducting polymers that lack ion-solvating or ionic functionality are typically poor ionic conductors, particularly in their electrochemically neutral state.
Additionally, OMIECs can be categorized as either heterogeneous or homogeneous. The primary difference between them is whether ionic and electronic transport occurs simultaneously throughout a single material (homogeneous) or if there is a separation between regions that primarily conduct ions and regions that primarily conduct electrons (heterogeneous). In the case of homogeneous materials, studies have shown that the efficiency of electrochemical doping by ions varies depending on the size of the ions and their compatibility with the side chains [7,8]. However, due to their complex microstructure and morphology, no such investigations have been reported for heterogeneous materials (Fig. 1).
This study aims to elucidate the electrochemical doping mechanism in heterogeneous materials, which remains poorly understood. By investigating the dependence of the performance of a heterogeneous OMIEC on ion size, we sought to observe ion dependency in the doping process. Through this approach, we clarified the doping process in poly (3,4-ethylenedioxythiophene) polystyrene sulfonate (PEDOT: PSS), the most widely used heterogeneous material.
2. METHODS
The films were fabricated following the methods in previous research [9,10]. Pristine PEDOT:PSS films were deposited by spin-coating on pre-cleaned substrates and annealed at 120 °C for 15 min after the aqueous PEDOT:PSS solution (Clevios PH1000, Heraeus) was filtered using cellulose acetate syringe filters (0.45 μm pore size, Advantec MFS, Inc.) prior to use. Pristine films were left in a bath of concentrated sulfuric acid (> 95%, Duksan Pure Chemicals) for 15 min, thoroughly rinsed with deionized water, and dried at 120°C for 15 min. Sulfuric acid treatment of PEDOT:PSS was conducted to enhance the μC* and stability of the OECT in an aqueous environment [9].
All electrochemical characterization were carried out using a potentiostat (Biologic, SP-200) connected with a three-electrode electrochemical cell. All PEDOT: PSS films were prepared on ITO substrates and were employed as the working electrode with a Ag/AgCl 3 M NaCl reference electrode and a platinum-coil counter electrode. Cyclic voltammetry (CV) measurements were carried out at a scan rate of 100 mV s−1. Electrochemical impedance spectroscopy (EIS) measurements were performed at the frequency range between 0.1 Hz and 100 kHz with a single sinusoidal signal of Eac=25 mV at various Edc levels.
All OECT devices were fabricated via the conventional photolithographic technique. Source and drain electrode patterns (Cr (5 nm)/Au (40 nm)) were prepared on a pre-cleaned glass substrates using a positive photoresist (GXR-601, Microchemicals GmbH). Channels were fabricated by depositing each film on the electrode-patterned substrate, followed by preparing a positive photoresist pattern and dry-etching the PEDOT: PSS films at the non-channel area. After the residual photoresist removal, the outer electrode patterns were passivated using SU-8 photoresist (Microchemicals GmbH). All electrical measurements were conducted using a Keysight B1500 precision semiconductor parameter analyzer while an Ag/AgCl reference electrode was employed as a gate electrode.
3. RESULTS AND DISCUSSION
To examine how ions influence the electrical behavior of PEDOT:PSS, OECTs were prepared with three 0.1 M chloride-ion-based aqueous electrolytes: NaCl, KCl, and ChCl (Choline chloride). These electrolytes differ in the crystallographic size of their cations (Fig. 2(b)). Fig. 2(d) shows the p-type transfer characteristics of a typical OECT measured in each electrolyte. To regulate the doping (oxidation) state of the channel, a voltage is applied to the gate electrode (VG). The conductance is then measured by applying a voltage of −0.6 V between the drain and source electrodes (VD) and recording the resulting drain current (ID). The ion size dependent changes in the maximum drain current (−ID, max) and threshold voltage (VT) for cation insertion were negligible (Fig. 2(c) and Fig. 2(d)). Since all the OECTs have an identical aspect ratio (W/L=1, W=80 μm, L=80 μm) and similar thickness (43 nm), we extracted the parameter (μC*) that characterizes the mixed ionic/electronic response of the polymer from equation (1). The extracted μC* product were 650, 644, 658 F cm−1 V−1 s−1 for Na+, K+ and Ch+ ions respectively (Fig. 2(c)). Therefore, no significant ion size dependence was observed even in the μC* product.
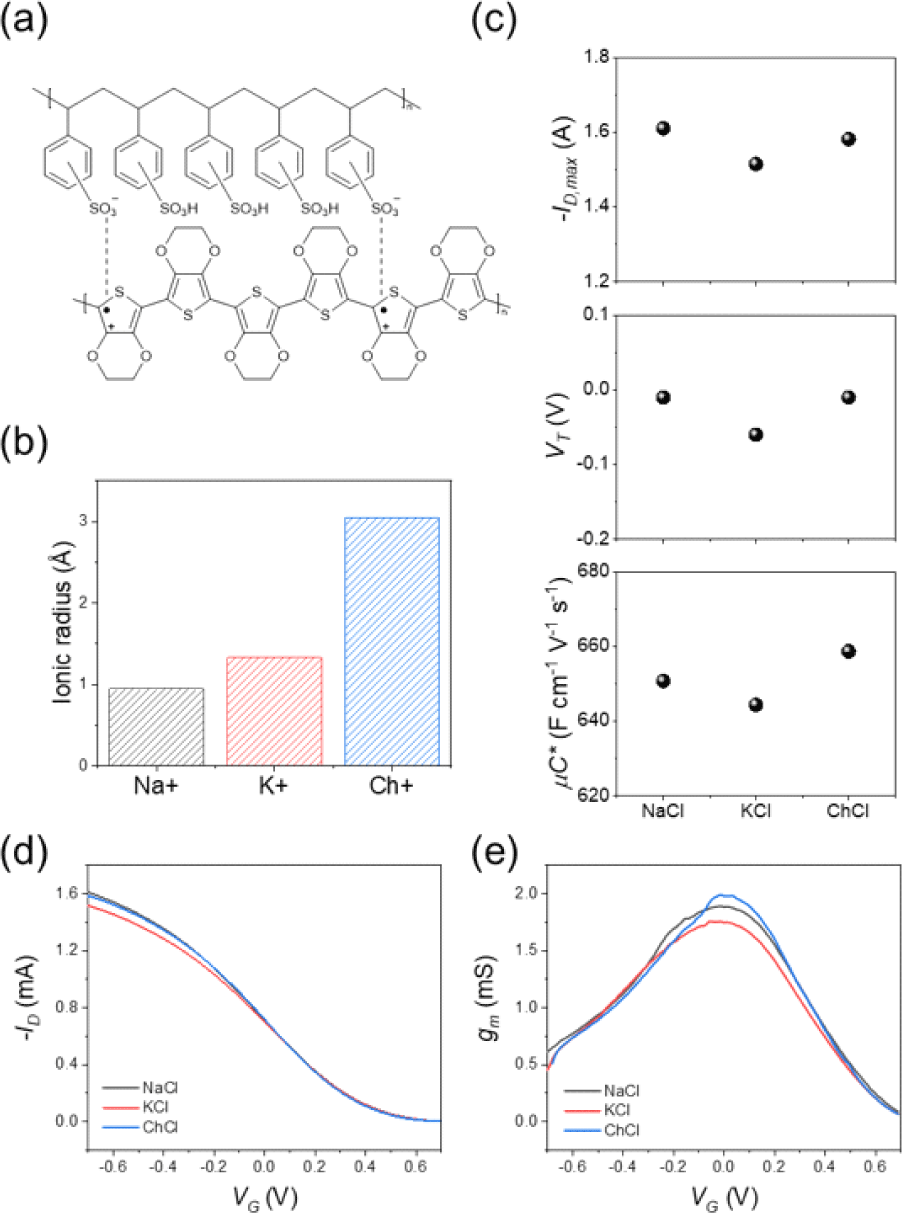
Volumetric capacitance was extracted by using the three electrode-based electrochemical impedance spectroscopy (EIS) to examine ion-mediated modulation. EIS data sets were analyzed with the Randles circuit, an equivalent circuit model composed of a serial resistor, parallel resistor, and parallel capacitor (Fig. 3(b)). C* increases with increasingly negative potentials reaching values of 104, 106, 100 F/cm3, at a potential of −0.4 V vs. Ag/AgCl for Na+, K+ and Ch+ ions respectively (Fig. 3(c)). Cyclic voltammetry (CV) experiments followed by EIS experiments were performed to measure the charge/discharge process of PEDOT:PSS. During the repeated charge/ discharge process, current is measured with applied potential at a scan rate of 100 mV/s (Fig. 3(d)). Clearly, the results of EIS and CV experiments suggest that electrochemical doping of PEDOT:PSS is ion size independent.
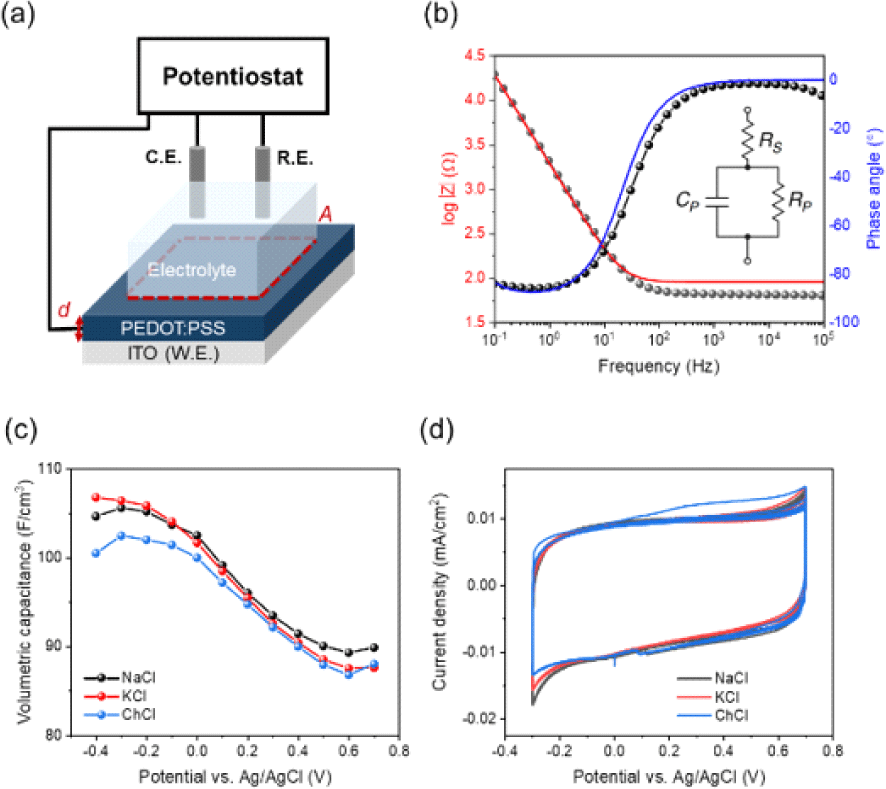
PEDOT:PSS is a polymer composite consisting of an electrically conducting π-conjugated polymer PEDOT and an ionically conducting polyelectrolyte PSS. In its natural doped state, positively charged holes in the PEDOT chains are compensated by negatively charged sulfonate () groups in PSS. Upon application of a positive gate bias, electrochemical dedoping of PEDOT occurs. During this process, the negatively charged sulfonate groups interact with cations from the dedopant instead of the PEDOT chains, leading to a reduction in carrier concentration and, a decrease in conductivity [11]. Since the proposed mechanism of doping/dedoping in PEDOT is directly related to the number of ionised/neutral PSS molecules, the influence of the cation variation is expected to be negligible.
Although no significant results were observed in transistor characteristics or electrochemical characteristics, there are still aspects worth investigating. One area to explore is the dependency of variation in pH on changes in the electrolyte, and its effect on transistor characteristics. The deprotonation of sulfonate groups by ion exchange decreases the pH, affecting PEDOT:PSS performance and OECT operation [12,13]. Investigating the cation dependence of this phenomena is expected to give further insight into the dedoping mechanism of PEDOT:PSS. Another avenue of research involves examining how changes in doping efficiency, influenced by the electrostatic force exerted by ions, may impact dynamic properties such as ionic mobility and transport [14]. To investigate this aspect, the effect of cation size on transient properties such as ion injection/ extraction time could be examined as a further study. Furthermore, while the current results indicate minimal ion dependence due to the dedoping process being primarily driven by cation-PSS binding, future studies could focus on anion dependence during the doping process. This would allow for a more detailed analysis of the direct interactions between PEDOT and anions (Fig. 4).
4. CONCLUSION
In conclusion, we conducted ion dependence experiments to understand the electrochemical doping process in PEDOT:PSS. For the figure of merit, μC*, of the OECT, it was observed that ChCl, the ion with the largest radius, exhibited a slightly higher value compared to other ions. Conversely, C* was found to be slightly lower. This might be due to improved alignment of PEDOT, which governs hole transport, resulting in a slight increase in mobility, while C* decreases marginally. However, no significant differences were observed. It is hypothesized that PEDOT, doped by PSS to form the hole transporting PEDOT:PSS, undergoes dedoping during electrochemical doping as cations interact with PSS. This interaction with PSS causes the dedoping of PEDOT:PSS, thereby not directly affecting the hole transport occurring in PEDOT.